Superconducting Magnets in Particle Accelerators
Introduction
Human curiosity has guided mankind to its greatest achievements. The old challenges though of mapping new territories and building transcontinental transportation have been met. Focus has now shifted and leads deeper into the infinitesimally small building blocks of life. Using the brute force of particle accelerators, we have been able to smash open these particles and write new chapters in our textbooks. For this exploration to continue though, we have to introduce new technologies to improve our methods. Superconductivity, one of the few perfections mother nature has given us, has found its place in particle accelerators. Through the use of superconducting magnets, we can now harness the power of these accelerators to their fullest extent.
Magnets In Accelerators
Without thinking of superconductivity an obvious question would be how magnets play a role in particle accelerators. We first must understand a beam is just a large amount of charge particles. During these accelerator experiments, the goal is to get as energetic and dense of a beam as possible onto your target. In order to do this we must focus and guide our beam. Through the introduction of two simple concepts, the Lorentz force and the Biot-Savart law, we can understand how magnets fill this role.
Guiding Beam
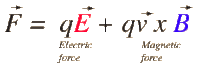
The Lorentz force tells us that inside a uniform magnetic field moving charge particles are deflected at right angles to the field. This will create a circular path, moving along the direction of the magnetic field. This can be thought of as beads on string. The string would represent the magnetic field while the beads are the particles. The beads are always “frozen” to the magnetic field moving in its direction. Now we must create the magnetic field. In order to do this we must introduce the Biot-Savart law. This simply states that a magnetic field will arise from a steady current. A common example is a wire with a current running through it. This will produce a magnetic field but not an efficient or effective one. A more useful geometrical setup for the current to run through is that of a solenoid. This geometry allows for a strong uniform and focused magnetic field. Often after acceleration particles must be refocused. This is due to the fact that they were not all moving in exactly the same direction before the acceleration. After this acceleration a properly placed solenoid magnet can allow for you to refocus our beam again. A perfect example of this setup is in Superconducting Linear Accelerator which will be discussed later. We do have a problem though. Looking back at the Lorentz force we see stress is put on the conductor. We first have these charged particles moving in a particle direction. When placed inside a magnetic field the force is then seen pointing out of the wire. This can be illustrated through the right hand rule. Experimentalists have found this strain on the conductor to be a real problem. While the beam can be successfully guided with these properties, we still need the ability to focus the beam. [4]
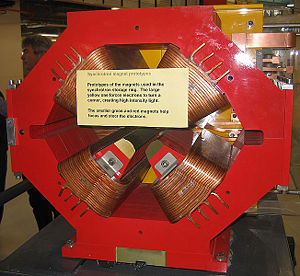
Focusing Beam
A common magnet used to achieve focusing is a wedge shape magnet. Particles farther out will be bent more into the focus of the beam, while closer in particles will be bent less. This is called weak focusing. Cyclotrons have this natural occurring in their design. Engineers who built these accelerators could not figure out why the beam would always align itself until they looked at the physics of weak focusing. Another class of focusing is called strong focusing. Quadrupoles are an excellent example in strong focusing. In this instance we use a focusing magnet followed by a gap then a defocusing magnet then another gap, repeating. When spaced correctly and used throughout a linear beam line, quadrupoles can be key to getting a precise high energy beam on your target. Though normal magnets can experience the necessary properties to guide and focus a beam, their limit in current density and magnetic field strength give rise to the search of a better alternative. While still displaying the same properties of normal magnets, superconducting magnets have proven themselves as a standard in new accelerator labs.
Superconducting Magnets
The result of superconductivity is zero resistance or no Ohms Law. The most appealing aspect of this is the magnet will no longer act as a resistor and lose energy in the form of heat. Intuition would seem to point out that a never ending current would save power. The only problem is we have to keep the magnet cool enough. The superconductor will only become superconducting at a certain critical temperature. This critical temperature is specific to every superconducting metal. In the end the power savings if any is not significant. The power cost will instead come from cooling the superconducting material to the required temperature. A commonly used coolant is liquid helium. Expensive and difficult to make, liquid helium is mostly used in the field of experimental academia. While higher temperature superconductors would only require inexpensive liquid nitrogen as a coolant, the metals which can achieve this are not as durable and easy to work with. The most popular material, and considered an industry standard, is niobium titanium for its good mechanical properties and durability. The construction of these magnets will be discussed later. The most significant advantage for experimentalist is superconducting magnets are able to obtain a much high current density than normal magnets.
High Current Density
Higher current density in the material will allow for much stronger magnetic fields. The more powerful accelerators require a larger magnetic field to focus the beam. Standard Iron magnets are limited to 2T while other materials such as copper may reach 100T for very short periods of time. The reason for this problem is heating. As stated before, normal magnets follow Ohm’s law in which electrical resistance in the magnet will cause heat. Superconducting magnets no longer have this problem. Superconducting magnets containing niobium can reach 6T-13T.These are much higher magnetic fields than any electrostatic magnet. Niobium tin magnets can achieve better current density but are not as durable and as a result more expensive to make. The construction of these superconductors is work intensive process.[6]
Construction
In the construction of superconducting magnets a pure metal is not forged. Instead strands of the desired material are wound together to make a wire. First thousands of niobium filaments are fused with bronze. This is then wrapped in niobium and jacketed in copper. The size of one wire is around 1-3mm. The outside is layered with glass insulation. After winding all the wires around a steel spool, the spool is placed into a furnace. The bronze then reacts with the niobium to make niobium tin. This process is used at the National High Magnetic Field Laboratory in which they continuously create the strongest continuous magnetic fields in the world. For a smaller accelerator lab the cost of using niobium tin has to be considered. The extra field strength is usually not cost effective enough to justify using it. If you are able to use liquid helium cooling, niobium titanium will achieve very adequate fields at a temperature far below the critical temperature. Once finished and put to use the superconducting magnets must be properly placed and cooled. If not, superconducting magnets can be dangerous.[7]
Quenching
As stated before in order to become superconducting the magnets must be cooled to the appropriate temperature. If not the magnetic has the potential to quench. Above this critical temperature the material behaves like a normal magnet in which the current or flow of electrons can be thought of as going through a crystal lattice. This lattice has many obstacles and the collisions will cause energy to be transferred in the form of heat. As more current is dumped the hotter the conductor gets. This temperature can get to the point of melting if not dealt with or cooled properly. When becoming superconducting we know we are able to obtain much higher current densities due to the properties of superconductors. If for some reason, due to eddy currents or too large a field, a small piece of the magnet heats up it can cause the rest of the magnet to heat resulting in a quench. This will put the magnet into a resistive normal state creating a large amount of heat. The energy of the magnetic field will also convert to heat releasing a sound of a whistle or even a bang. Damage to the magnet is rare unless the current density is extremely high. The real danger is asphyxiation. For example in a small room that contains an MRI machine, the superconductors are cooled with liquid nitrogen. In the event of a quench the liquid nitrogen will quickly turn to liquid gas and possibly push out and remove all the oxygen. Most areas containing superconducting magnets have regulation for the ventilation systems. At CERN a series of superconducting magnets quenched due to faulty part manufacturing. Explaining what superconductors are, their advantages, and how they are made is critical to their understanding. A detailed example of an actual accelerator using all these concepts together will help piece together all the information.[8][9]
Superconducting Linear Accelerators
Superconducting Magnets have the role of focusing and guiding the beam in accelerators. Other superconducting applications are found in accelerators in conjunction with superconducting magnets. An excellent example of this is the popular superconducting linear accelerator as mentioned before. In this instance the use of superconductors is to both focus the beam and accelerate it. The focusing will be done by the superconducting solenoid magnets and the accelerating is achieved by the superconducting niobium resonators. To describe a common setup for this type of accelerator you would have the following. First a cryostat houses four resonators and 2 solenoids. The linear order of the parts is two resonators one solenoid and then two resonators and one solenoid. After the first cryostat more cryostats can be placed one after another. For this particular type of accelerator to work properly the particles cannot come in continuously but instead in bunches or packets. Imagine this bunch approaching one end of a resonator which looks like a broken rollercoaster loop as depicted in the picture. If the particles were positively charged a negative charge would be on the first end of the resonator. The packet is attracted to the opposite charge. Once the bunch gets half way through the center of the resonator, the polarity on both ends of the resonator switches. This pushes the bunch again as it is pushed from behind and attracted forward. This process happens again and then the particles enter the superconducting solenoid. The shape of the solenoids magnetic field focuses the beam which is an excellent thing to do after accelerating particles. The reason for this is the particles in the bunch may not have all been moving in the exact same direction. The acceleration will exploit this and spread the beam line out. Once the bunch enters the solenoid it is focused before in goes into another resonator. How does superconductivity play a role in this? The magnets as we already know are superconducting and focus the beam. But, the niobium resonators are super conducting as well. Their goal though is not to supply a uniform magnetic field but instead a constantly changing polarity between both ends. This is where the current comes in. Controlled by and RF frequency the current changes the polarity on both ends of the resonator around 97million times per second. An added feature to most of these resonators is a persistent switch. This switch is a small piece of superconducting wire which heats up and can set the current on the superconductor. Once the desired current density has been reached the wire becomes superconducting again and the resonators current flows without resistance. The advantage of this is the isolation of the resonator as stated before. The liquid helium surrounds parts of the resonator and less helium boiling will occur.[10][11]
Conclusion
Superconducting magnets have allowed for the advance in the field of high energy physics, nuclear physics, and magnetic resonance imaging. These MRI machines in hospitals have allowed for new medical procedures as we can see where we were never able to see before. One of the major challenges ahead for superconducting magnets will be finding a durable high temperature superconductor. As we move to a more energy demanding world, superconductors will play a larger role in the success of our species.
References
[1] Science News, World's Largest Superconducting Solenoid Magnet Reaches Full Field, 2006 http://www.sciencedaily.com/releases/2006/09/060925075001.htm
[2] World culture pictorial, WcP.Scientific.Mind's blog, 2011 http://www.worldculturepictorial.com/blog/blogs/wcpscientificmind?page=5
[3] Hyperphysics, Magnetic Force, 2011 http://hyperphysics.phy-astr.gsu.edu/hbase/magnetic/magfor.html
[4] CERN, Superconducting magnet technology for particle accelerators and detectors, 2006 http://cdsweb.cern.ch/record/1036201
[5] Find target Reference, Quadrupole magnet, 2011 http://reference.findtarget.com/search/Quadrupole%20magnet/
[6] Hyperphysics, Superconducting Magnets, 2009 http://hyperphysics.phy-astr.gsu.edu/hbase/solids/scmag.html
[7] National High Magnetic Field Laboratory, Making Superconducting Magnets, 2004 http://www.magnet.fsu.edu/education/tutorials/magnetacademy/superconductingmagnets/page5.html
[8] American Magnetics, Characteristics of Superconducting Magnets, 2011 http://www.americanmagnetics.com/charactr.php
[9] Big Science News, LHC Quench Stops CERN: Re-start Delayed Again, 2008 http://bigsciencenews.blogspot.com/2008/09/lhc-quench-stops-cern-re-start-delayed.html
[10] Florida Sate University Physics, Superconducting Linear Accelerator Laboratory, 2000 http://www.physics.fsu.edu/Nuclear/Brochures/SuperconductingLinearAcceleratorLaboratory/
[11] Argonne Tandem Linear Accelerator System, The ATLAS Facility, 2003 http://www.phy.anl.gov/atlas/facility.html
- ↑ http://www.sciencedaily.com/releases/2006/09/060925075001.htm
- ↑ http://www.worldculturepictorial.com/blog/blogs/wcpscientificmind?page=5
- ↑ http://hyperphysics.phy-astr.gsu.edu/hbase/magnetic/magfor.html
- ↑ http://cdsweb.cern.ch/record/1036201
- ↑ http://reference.findtarget.com/search/Quadrupole%20magnet/
- ↑ http://hyperphysics.phy-astr.gsu.edu/hbase/solids/scmag.html
- ↑ http://www.magnet.fsu.edu/education/tutorials/magnetacademy/superconductingmagnets/page5.html
- ↑ http://www.americanmagnetics.com/charactr.php
- ↑ http://bigsciencenews.blogspot.com/2008/09/lhc-quench-stops-cern-re-start-delayed.html
- ↑ http://www.physics.fsu.edu/Nuclear/Brochures/SuperconductingLinearAcceleratorLaboratory/
- ↑ http://www.phy.anl.gov/atlas/facility.html